Contents
1. Why bother?
Alabaster dissolution rate measurements can strengthen the scientific basis of your passive sampler calibrations. Passage over the water boundary layer is one of the steps in the accumulation of compounds by passive samplers. Knowledge of its resistance is relevant because the accumulation rates of a passive sampler can never be larger than what is permitted by the boundary layer. It answers questions such as
- Will increasing the water flow velocity speed up the sampler-water exchange kinetics?
- If so: by how much?
- How can I relate stirring or pumping rates to flow velocities at my monitoring sites?
Even if no flow effects are expected: it can be good to verify and know for sure.
2. Alabaster method: qualitatively
Alabaster is a natural deposit of calcium sulfate dihydrate. It is moderately soluble in water (~2.5 g/L in freshwater and ~ 6.5 g/L in seawater). When you put a piece of alabaster in water, it starts to dissolve. The calcium sulfate concentration at the surface is saturated and far away from the surface the concentration is lower (close to zero). The alabaster dissolves fast when the water flow velocity is high.
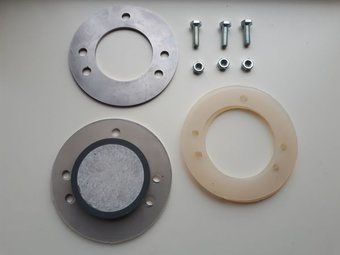
When you measure the mass loss rate, then you know something about how easily calcium sulfate moves through the water boundary layer at the surface. But the mass loss rate also depends on the concentration difference. For example in seawater the solubility is higher than in freshwater, so you have a larger concentration difference between the surface and bulk water in that case. We divide the mass loss rate by this concentration difference to account for that. The mass loss rate (grams per day) divided by the concentration difference (grams per liter) gives something with the unit liters per day.
Hey! that sounds like a sampling rate. In fact: it is a sampling rate. It is the rate at which the water samples the saturated solution at the alabaster surface. It is equal to the sampling rate as we know it in passive sampling methods. But: for the case when the transport is completely controlled by the water boundary layer. So the alabaster method gives you the sampling rate for water boundary layer controlled kinetics. (There are some differences related to the differences in diffusion coefficients; see section 3.)
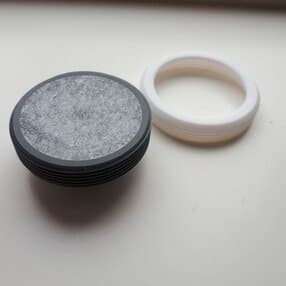
Compare the sampling rate from the alabaster method with the sampling rate for organic compounds (or metals, nutrients,…) and you know immediately to what extent your sampling rate is controlled by the flow.
It is often convenient to divide the sampling rate also by the surface area, to make it independent of the size of the plate. Then you get the mass transfer coefficient. This has the units of a velocity (dm per day, micrometer per second, or other). Mass transfer coefficients are useful if you want to go deeper into the modeling of passive samplers. If you feel uncomfortable with mass transfer coefficients: think of them as surface area normalized sampling rates.
3. Alabaster method: quantitatively
When you put a piece of alabaster in water then the water right at the surface is saturated with calcium sulfate. The higher concentration at the surface drives the flux to the surrounding water, and the plate loses weight. When the water volume is infinitely large, then the mass loss (Δm) after some time (t) equals
Δm = kw A C* t
where kw is the mass transfer coefficient of the water boundary layer, A is the area of the plate, and C* is the solubility of the alabaster in the water. To obtain kw (or Rs = kwA) you measure the mass loss and the exposure time, and calculate the solubility. When the water volume is small, then the buildup of calcium sulfate in the water should be taken into account. No worries about solubility calculations and concentration buildup: PaSOC’s calculation template takes care of that. For details see references below (Opdyke et al. 1987; Santshi et al. 1991; O’Brien et al. 2011; Booij et al. 2017).
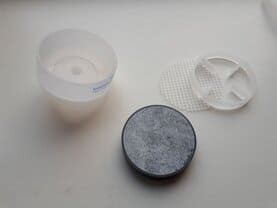
Now you have the kw of calcium sulfate. This is not exactly the same as the kw of an organic compound because the diffusion coefficient (Dw) of calcium sulfate is a bit larger than the Dw of an organic compound (8×10-10 m2/s versus typically 5×10-10 m2/s at 20 °C). Several decades of engineering literature suggests kw ~ Dw2/3 , which means that the kw of an organic compound is 1.4 times smaller than of calcium sulfate. No worries about estimating Dw of calcium sulfate and organic compounds: the calculation template takes care of that if you want.
4. In practice
Get kw as follows
- Weigh the plate with 0.1 mg precision.
- Expose for 20 min (high flow) to 2 h (low flow), aiming for a 200-400 mg mass loss.
- Dry at 40 °C in an oven
- Cool down under ambient conditions
- Weigh again
- Fill out the calculation template
- Copy the kw of calcium sulfate and/or kw of your the target compound. Multiply by the surface area to get Rs for water boundary layer controlled kinetics. For use with POCIS: use the surface area that is covered by the sorbent. Present best guess is that ~25% of the membrane area is covered by the sorbent (POCIS with 54 mm membrane diameter and 220 mg sorbent).
5. Limitations
Flow limitation: At very low flow velocities (below ~ 2 cm/s) the dissolution process generates its own current. The density of a saturated calcium sulfate solution is 0.2% larger than the density of water. At low flow this solution can sink down due to its higher density, and draws solute free water over the plate.
Exposure time limitation: If the exposure time is too long, then the final solution is completely saturated with calcium sulfate, or the plate is completely dissolved. In either case information on the kinetics is lost. A mass loss of 200 to 400 mg is optimal. Smaller values cause a decrease in weighing accuracy, and larger values reduce the number of measurements that can be made with each plate.
The method is fast and easy to apply at low cost. This is both its strength and its weakness. For time-integrative kw measurements over time periods of days and weeks other methods should be used.
6. Alabaster plates and Passive Flow Monitors
Passive Flow Monitors (PFMs – O’Brien et al. 2009) were developed for measuring time-averaged flow velocities near passive samplers. Both alabaster plates and PFMs are based on the dissolution of calcium sulfate dihydrate. That is where the similarity ends.
- Alabaster is a nonporous rock-like material. The method is firmly rooted in engineering science and hydrodynamics. It is used to measure kw. Typical mass losses are approximately a few hundred milligrams. Deployment times are 0.3 to 2 h.
- PFMs consist of a gypsum plaster cast in a plastic tube. The mass loss rate of the cast is correlated empirically with the external flow velocities. Typical mass losses are 5 to 40 g. Deployment times are approximately two weeks.
Both methods have their own niche of application, and answer different questions. In short: PFMs are used to measure average flow velocities, and alabaster plates are used for measuring mass transfer coefficients of the water boundary layer.
7. How to order
All alabaster plate assemblies are custom made, to fit your specific housing design, as there is little standardization of passive sampler holder designs. Standard thickness is 10 mm. Smaller thickness can be made if you like. The plates are fitted in PVC for protection against physical damage. All plates come with a kw calculation template. Download the manual here. Prices range between EUR 120 and EUR 160, depending on the sampler housing. Contact keesbooij@pasoc.eu for a quotation or for questions.
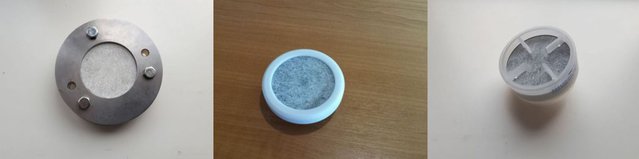
8. Further reading
Booij, K., Maarsen, N.L., Theeuwen, M., van Bommel, R., 2017. A method to account for the effect of hydrodynamics on polar organic compound uptake by passive samplers. Environ. Toxicol. Chem. 36, 1517–1524. https://doi.org/10.1002/etc.3700
O’Brien, D.S., Booij, K., Hawker, D.W., Mueller, J.F., 2011. Method for the in situ calibration of a passive phosphate sampler in estuarine and marine waters. Environ. Sci. Technol. 45, 2871–2877. https://doi.org/10.1021/es101645z
O’Brien, D.S., Chiswell, B., Mueller, J.F., 2009. A novel method for the in situ calibration of flow effects on a phosphate passive sampler. J. Environ. Monit. 11, 212–219. https://doi.org/10.1039/B809901D
Opdyke, B.N., Gust, G., Ledwell, J.R., 1987. Mass transfer from smooth alabaster surfaces in turbulent flows. Geophys. Res. Lett. 14, 1131–1134. https://doi.org/10.1029/GL014i011p01131
Santschi, P.H., Anderson, R.F., Fleisher, M.Q., Bowles, W., 1991. Measurements of diffusive sublayer thicknesses in the ocean by alabaster dissolution, and their implications for the measurements of benthic fluxes. J. Geophys. Res.-Oceans 96, 10641–10657. https://doi.org/10.1029/91JC00488